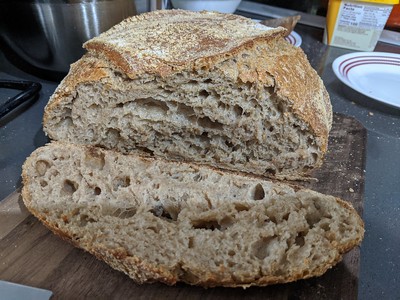
Greetings,
I hope you're all staying healthy, well fed, employed, and finding ways to stay connected.
This month I am tackling science articles discussing the relationship between COVID-19 and conservation (plus a couple related ones on air quality). It's not a representative sample of the literature. I drew from 1) articles either sent to me directly or 2) articles cited (in email or twitter or blogs I ran across) to support high-level conclusions, and I reviewed the ones that seemed both the most relevant and relatively high-quality.
I also don't think I'm qualified to weigh in. But the topic is unavoidable, and I would rather make the attempt than ignore it. I was prompted partly by Bob Lalasz' excellent post "The Wrong Kind of Serenity," even though I don't know if my expertise is sufficient to the task.
Most of the blogs and emails I've seen on this topic either seem to be in support of a clear agenda, or were written for a constrained audience and can't be shared. I especially welcome your feedback and perspectives on both this summary and the papers themselves. Please also send other papers & resources you have found the most useful (or problematic).
Overall, I didn't find a lot of consensus conclusions and recommendations. But there is agreement that the more humans (and our domesticated animals) live near to nature (especially when primates and bats are present), spend time in nature, and convert wildlife habitat (for settlements or food production), the more chance there is of being exposed to zoonotic disease (spread between animals and humans). It is also fair to say that reducing air pollution will have strong benefits to human health even if the potential link between COVID-19 mortality and poor air quality is not supported by additional research.
Finally, there is a lot of debate about whether higher biodiversity reduces the risk of disease transmission. The basic idea is that with more species there will be fewer compatible hosts for any given species. On the other side, as biodiversity declines some wildlife species prone to harboring zoonotic disease will be lost. I didn't read enough of this literature to come to an informed conclusion, but I'm not yet convinced. You can read a bit about it here (this article favors the idea that biodiversity loss worsens disease risk but includes a counterpoint): https://www.caryinstitute.org/news-insights/media-coverage/more-we-lose-biodiversity-worse-will-be-spread-infectious-diseases
If you know someone who wants to sign up to receive these summaries, they can do so at http://bit.ly/sciencejon
CONSERVATION & HUMAN HEALTH:
DISEASE TRANSMISSION:
Smith & Guégan 2010 is a (long) summary of the origin and location of all human pathogens. It's useful context for thinking about COVID-19 and other zoonotic diseases. For emerging infectious disease in particular (diseases which are new or becoming more significant), about 3/4 of them are zoonotic. They also cite an earlier paper (Woolhouse & Gowtage-Sequeria 2005) that ranks the drivers of emerging pathogens (by # of pathogen species, NOT by impact), which from most to least spp. are: "(1) changes in land use or agricultural practices, (2) changes in human demographics and society, (3) poor population health, (4) hospital and medical procedures, (5) pathogen evolution, (6) contamination of food supplies or water sources, (7) international travel, (8) failure of public health programs, (9) international trade, and (10) climate change." Land-use change has been more key for bacterial and zoonotic disease, and mostly involves people coming into closer contact with nature and wildlife.
Morse et al. 2012 offers ideas from the past to predict and prevent the next zoonotic pandemic. It has a useful summary of how these pandemics emerge (see Panel 1), and Figure 1 has a global risk map which could inform monitoring. However, we have never predicted a pandemic before humans became infected. They recommend continued improvement of global monitoring to quickly identify novel pathogen outbreaks, while noting this has been a top recommendation for decades. Other suggestions seem impractical (better sanitation and biosafety practices in potential hotspots) or too expensive and complicated. For example, modeling which wildlife species are most likely to harbor emerging zoonoses (zoonotic diseases) would enable better monitoring. But there are thousands of potential pathogens to evaluate. The PREDICT program (from USAID) is listed as an example of a successful approach (combining collecting samples from wildlife and identifying the pathogens posing the most potential risk). Ironically the program ended fieldwork in September 2019, and was ended in March 2020 before being given an emergency 6-month extension in April 2020. It will be interesting when later analysis reveals whether or not they had the data needed to predict the emergence of SARS-CoV-2 / COVID-19.
I skimmed quite a few articles on the "dilution effect" arguing that higher biodiversity leads to lower disease risk before settling on Ostfeld & Keesing 2012 to review here. I like that they are methodical in exploring the issue, but it's a long article. I found the first half fairly unconvincing: modeling 'adding diversity' yields different results than much more likely cases of losing diversity, and the agricultural example doesn't apply well to zoonoses. But starting with p10 of the PDF (p166) there are useful case studies showing that diseases w/ generalist animal hosts like rodents will tend to be higher risk as diversity goes down. But despite showing biodiversity loss can raise disease risk, on p19 of the PDF (p175) they cite a metaanalysis finding that species richness had very little effect on zoonotic disease emergence. Instead human population density was the key factor. I look forward to reading more on this topic to have a more informed opinion.
Johnson et al. 2020 is a timely analysis of which mammals have the most potential to transmit disease to humans (estimated by the number of zoonotic virus species, an imperfect but useful proxy). Overall, the more common a species was, the more viruses they shared with humans. Domesticated mammals (12 species) were the highest-impact variable in their model, and they hosted 50% of all zoonotic virus species (not necessarily exclusively). 75% of virus species were hosted by either rodents, bats, and/or primates. Primates and bats host more viruses per mammal species, and bats in particular have traits that make transmission to other species more likely (rodents are significant partly because there are many common rodent species who live near humans). Finally, while they note that among threatened species virus richness goes up when the mammals are threatened by habitat loss, those species still have fewer virus species than more common mammals.
Faust et al. 2018 models how different rates and amounts of habitat loss impact the risk of zoonotic disease. The primary finding is intuitive: risk is fairly low when habitat loss is either very low (few humans in contact w/ nature) or very high (few wild populations in contact w/ people). So it's the mix of humans and natural habitat that poses more risk. In general, faster land conversion reduces exposure and thus risk. However, they note that fast conversion can also rarely lead to the largest outbreaks (where a lot of displaced species interact with a large pool of human hosts who are likely to mix with other humans). Figure 2 has interesting case studies of zoonotic diseases with different transmission modes, and Figure 5 shows how infection rates vary over time depending on rate of habitat loss.
Bloomfield et al 2020 asks what factors are associated with physical contact between humans and wild nonhuman primates (and thus potential concerns with disease exposure). They looked at smallholder farmers in Uganda living near forest patches (which they call "core") in an area with ongoing deforestation to create new farms and pastures (which along with settlements they call "matrix"). The results are not surprising: people who go to forests (for hunting & foraging for food, and/or gathering small trees for construction) or live in areas with more forest fragmentation have slightly higher chances of contacting primates.
Mills et al. 2010 summarizes the scant information on how climate change can affect zoonotic disease. They list four ways climate change can impact vector-borne zoonotic disease via changes to the host and/or vector: range shifts that result in contact w/ new human populatoins, changes in population density (leading to more or less human contact), changes in prevalence of infection (leading to more or less contact w/ infection), and changes in pathogen load (leading to more or less chance of transmission per contact). They list case studies of each, and note that while there is evidence of climate change having increased risk, there are many confounding variables not accounted for. For example, despite the mosquito host of Dengue and Zika becoming established in Texas, the diseases remain relatively rare despite nearby epidemics in Mexico (the difference may be due to more air conditioning lowering exposure in Texas). They close with a research agenda for the kinds of studies most needed to better understand how climate will impact zoonotic disease.
How does migration impact the risk of zoonotic disease? Altizer et al. 2011 find that it's complicated. Migrations can spread pathogens including to other species. They can also increase risk via reducing host immune function, and increasing exposure to pathogens for the migratory species. But migrations can also cause disease risk to go down by leaving parasites behind (and making it harder for parasites to reproduce in their absence), or removing infected animals from the population (since they're not fit enough to migrate, which may also provide selection pressure favoring less virulent pathogens). On net, migration may be bad for specialist pathogens, parasites that build up over time, pathogens transmitted via biting vectors or intermediate hosts, and pathogens transmitted mainly from adults to juveniles during breeding. Migration may help generalist parasites where there are shared stopover areas or wintering grounds, or help specialist pathogens that spread better with dense populations common during migration.
AIR QUALITY:
While poor air quality is a leading global health risk, I've only seen one study so far directly looking at how it impacts COVID-19 mortality (and it's a preprint, so it hasn't been peer-reviewed yet). Wu et al. 2020 looked at correlation between long-term U.S. air quality (specifically PM2.5: tiny particles < 2.5 μm in diameter) and found that fairly small increased in PM2.5 concentration (1 μg/m3) were associated with a 15% increase in COVID-19 death rate (compared to a 0.7% increase in the rate of all-cause mortality). They control for quite a few confounding variables (e.g. hospital beds, population, obesity, smoking, poverty, etc.), but note that limited testing means they can't properly control for outbreak size (which could be the primary driver of their results). Initial discussion has identified some other missing variables (like accounting for respiratory diseases like COPD or lung cancer), but this is still a useful data set to inform discussions and more research. The authors are also report they are publishing similar results for China and Italy. It's not clear whether short term improvements in air quality from the lockdown would make any difference. There are interesting comments on the initial version of the paper.
Zhang et al. 2019 shows how many lives can be saved by reducing air pollution, using an initiative in China (2013-2017) as a case study. The authors estimate that "national emissions of SO2, NOx, and PM2.5 decreased by 59%, 21%, and 33%, respectively." This reduction in PM2.5 (by ~20 μg/m3) avoided ~410,000 premature deaths. They have lots of detail about all the actions that made this possible, and Fig 4 shows how much each change contributed to avoided deaths; the biggest contributions came from stronger industrial emissions standards and upgrades to industrial boilers. Note that this study was published pre-COVID-19, so doesn't include potential benefits of reduced complications from the disease (although even the reduced levels in China are still much higher than the US).
Tessum et al. 2019 is a very short but useful look at racial inequity of PM2.5 air pollution. It is also a great overview of sources and risks of PM2.5 (see Fig 1), which they note is responsible for about 2/3 of US deaths from environmental causes. But their key finding is that black and Latinx people are exposed to 56% and 63% more PM2.5 than the relative amount of pollution caused by the goods and services they consume. Conversely, non-Latinx white people & other races (they lump whites with Asians, Native Americans, and all other races) are exposed to 17% less PM2.5 relative to their consumption. From 2003-2015, overall PM2.5 exposure dropped ~50% on average, while inequity decreased for black people but remained similar for others. Given the findings of the Wu 2020 preprint (that PM2.5 exposure increases COVID-19 mortality), this disease could further racial inequity. However, to date the CDC has found that while COVID hospitalized patients are disproportionately black, that there are fewer Latinx patients than in surrounding communities, so there are clearly other factors at play.
REFERENCES:
Altizer, S., Bartel, R., & Han, B. A. (2011). Animal Migration and Infectious Disease Risk. Science, 331(6015), 296–302. https://doi.org/10.1126/science.1194694
Bloomfield, L. S. P., McIntosh, T. L., & Lambin, E. F. (2020). Habitat fragmentation, livelihood behaviors, and contact between people and nonhuman primates in Africa. Landscape Ecology, 35(4), 985–1000. https://doi.org/10.1007/s10980-020-00995-w
Faust, C. L., McCallum, H. I., Bloomfield, L. S. P., Gottdenker, N. L., Gillespie, T. R., Torney, C. J., … Plowright, R. K. (2018). Pathogen spillover during land conversion. Ecology Letters, 21(4), 471–483. https://doi.org/10.1111/ele.12904
Johnson, C. K., Hitchens, P. L., Pandit, P. S., Rushmore, J., Evans, T. S., Young, C. C. W., & Doyle, M. M. (2020). Global shifts in mammalian population trends reveal key predictors of virus spillover risk. Proceedings of the Royal Society B: Biological Sciences, 287(1924), 20192736. https://doi.org/10.1098/rspb.2019.2736
Mills, J. N., Gage, K. L., & Khan, A. S. (2010). Potential Influence of Climate Change on Vector-Borne and Zoonotic Diseases: A Review and Proposed Research Plan. Environmental Health Perspectives, 118(11), 1507–1514. https://doi.org/10.1289/ehp.0901389
Morse, S. S., Mazet, J. A. K., Woolhouse, M., Parrish, C. R., Carroll, D., Karesh, W. B., … Daszak, P. (2012). Prediction and prevention of the next pandemic zoonosis. The Lancet, 380(9857), 1956–1965. https://doi.org/10.1016/S0140-6736(12)61684-5
Ostfeld, R. S., & Keesing, F. (2012). Effects of Host Diversity on Infectious Disease. Annual Review of Ecology, Evolution, and Systematics, 43(1), 157–182. https://doi.org/10.1146/annurev-ecolsys-102710-145022
Smith, K. F., & Guégan, J.-F. (2010). Changing Geographic Distributions of Human Pathogens. Annual Review of Ecology, Evolution, and Systematics, 41(1), 231–250. https://doi.org/10.1146/annurev-ecolsys-102209-144634
Tessum, C. W., Apte, J. S., Goodkind, A. L., Muller, N. Z., Mullins, K. A., Paolella, D. A., … Hill, J. D. (2019). Inequity in consumption of goods and services adds to racial–ethnic disparities in air pollution exposure. Proceedings of the National Academy of Sciences, 116(13), 6001–6006. https://doi.org/10.1073/pnas.1818859116
Woolhouse, M. E. J., & Gowtage-Sequeria, S. (2005). Host range and emerging and reemerging pathogens. Emerging Infectious Diseases, 11(12), 1842–1847. https://doi.org/10.3201/eid1112.050997
Wu, X., Nethery, R. C., Sabath, B. M., Braun, D., & Dominici, F. (2020). Exposure to air pollution and COVID-19 mortality in the United States. MedRxiv, 2020.04.05.20054502. https://doi.org/10.1101/2020.04.05.20054502
Zhang, Q., Zheng, Y., Tong, D., Shao, M., Wang, S., Zhang, Y., … Hao, J. (2019). Drivers of improved PM 2.5 air quality in China from 2013 to 2017. Proceedings of the National Academy of Sciences, 116(49), 24463–24469. https://doi.org/10.1073/pnas.1907956116
Sincerely,
Jon